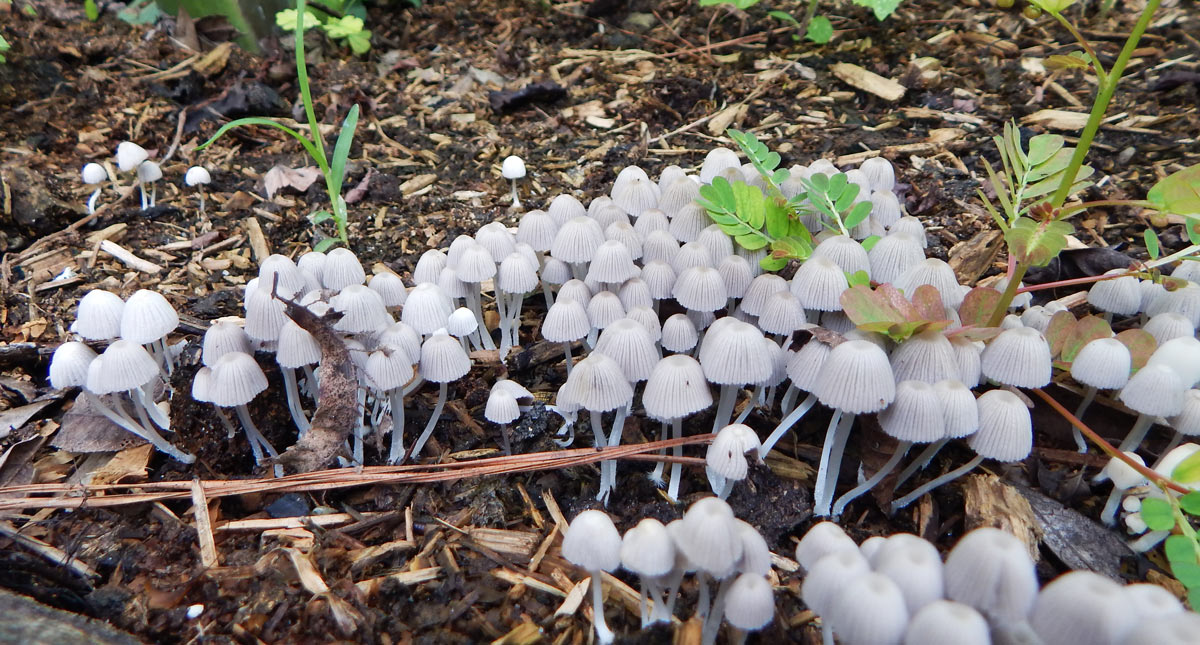
Introduction to Mushrooms and Mycology by Danny Miller, education@psms.org
Hunting for mushrooms can be a very rewarding hobby, not just for edibles but for the wide array of colours, shapes and odors that they come in. You might find mushrooms that are bright red, purple, green or almost any colour of the rainbow. They might smell of sweet almonds, black licorice, grape bubble gum or garlic. Almost immediately after beginning to mushroom hunt I was finding fascinating species very easily and wondering “Am I just lucky today or have these always been here and I just haven’t noticed?” This is a common story. The woods are full of the most amazing things that are getting overlooked all the time because we’re so caught up in our own little world. All it takes is a moment to look around to start discovering and being inspired by the fascinating world of fungi.
I regret I cannot demonstrate the odors on this web page.
Learning to Identify
People ask me all the time how to quickly tell which mushroom is which. All of the books describe how important it is to spend a few hours getting a spore print of a gilled mushroom, and carefully comparing dozens of different characters to make sure they all match your mushroom before coming to any conclusions. You might spend all day keying out a mushroom and coming to a tentative conclusion, and then go to hand it to an experienced identifier and from across the room they will tell you what it is. How did they do that? They didn’t have any time to examine the mushroom and determine if the cap was scaly or only fibrillose or if the stem was pruinose or not, and they certainly didn’t take a spore print. This is because the experienced identifier has learned to recognize the mushroom as you learn to recognize your friends and family. When cousin Steve walks up to you, you don’t think “Ah, mid-length black hair, glasses and freckles, age range 40-49, that’s cousin Steve”. You have synthesized everything in your mind about Steve that makes Steve Steve to the point where you can just recognize him. This will eventually happen to you for certain mushrooms. You can probably already identify a store bought Safeway brown button mushroom without thinking about it too much. That does not mean that you shouldn’t take the time to make spore prints and carefully go through mushroom keys. You cannot learn to recognize a mushroom by only reading about it, you have to play with it and look at it carefully while answering questions about it to really get to know it. Just like you didn’t really get to know cousin Steve by looking at his picture in the family album, you got to know him because he came to visit every year for the holidays. So when you ask the identifier how they could tell what the mushroom was, and they say “I’m not sure, it’s hard to explain”, they are not being mean. It’s just as if somebody asked you to describe how to recognize cousin Steve because they need to pick him up at the airport – it would be hard for you to describe Steve in a way that would allow somebody else to pick him out of a crowd.
One of the most important things a mushroom book or website can do is help you tell mushrooms apart from each other. A technical monograph will describe many mushrooms in extreme detail, but that’s not enough. You might have to read several pages of notes on two species and take notice yourself about what the differences are (much might be few). The next step is to realize which of those differences are important and which aren’t. The best criteria are those which are easily noticeable and reliably different between the two mushrooms. I think the most useful part of a guide book is the section that talks about the mushroom and its close lookalikes, like the “Comments” section in Mushrooms Demystified or the “Notes” and “Similar” sections of the MatchMaker program. That is what I have tried to do on these pages – focus on the unique characters of each mushroom that allow you to most quickly tell them apart, and I have placed photos of all the similar species side by side for easy comparison, unlike the typical guide book which often lists them in alphabetical order.
Something that is very important to take note of if you are going to try and identify a mushroom is… did the mushroom sprout out of the ground, or is it growing out of a piece of wood? There are two main ways that mushrooms get nutrition and figuring that out can be an important part of identifying it.
First of all we have to talk about what a mushroom really is. Fungi are organisms that are different from both plants and animals, although we used to think they were a kind of plant (because they are attached to the ground and can’t wander around freely). But it turns out that genetically, fungi are closer to animals than they are to plants – we both have chitin in our cell walls, for instance. The actual fungus grows as a network of threads called mycelium that permeate the ground and can grow for miles, sort of like the roots of a plant but smaller than the width of a human hair. When conditions are right, and the fungus feels it has a good chance at reproducing, it will expend the energy to grow a mushroom (like a fruit or a flower that a plant grows). Because of the vast difference in size between the invisible threads of the fungus itself and its fruit, the mushroom, it almost seems analogous to a tree growing an apple that is the size of the Empire State Building. Unlike plants that sprout flowers and fruit like clockwork every year, not all fungi will grow mushrooms every year. Since the organism is invisibly tiny, you can imagine that it takes a LOT of energy to create a “fruit” that is orders of magnitude more massive than itself, so they are fussy about when they fruit. Nobody understands fully what triggers them. Some mushrooms are only seen to sprout once every ten or twenty years, while others come up reliably several times a year. It has something to do with the temperature and humidity and soil acidity being ideal, but “ideal” is different for different fungi. So you might say that while the millions of species of plants and animals all look different, and you can tell them apart fairly easily, the millions of species of fungi all look almost identical to the naked eye (invisibly small thread networks) but their fruits all look different. So when we study mushrooms, we are studying the different fruiting bodies of different fungi, not the fungus itself. Many fungi never make fruiting bodies big enough to see very well. For instance, the mold Penicillin is just a thin layer of fuzz, and some species are much smaller than that. Most mushroom clubs, mushroom books and mushroom pages like this one are mostly concerned with those fungi that make large fruiting bodies that you are likely to notice (and care about). But there are many more thousands of closely related species that go mostly unnoticed because no part of them ever gets big enough to get your attention.
You will see tiny mushrooms almost all year round (e.g. Mycena) but the larger, fleshier fruit bodies mostly fruit during certain times of the year because they take a lot more energy to produce and perhaps the fungus is being fussier about when to sprout, wanting to make sure the conditions are right.
Some mushrooms are mycorrhizal, meaning that they live in a symbiotic relationship with trees and other plants. Their mycelium actually grows in with the network of tree roots. Remember back in grade school when you learned that plants make their own food using the chlorophyll that makes them green to turn sunlight into sugar? Well, that’s not the whole story. If the tree only ate sugar it would be as unhealthy as you or I living on an all candy diet. It turns out the mushroom’s thin mycelium are very good at getting vitamins and minerals out of the soil, but plant roots are not. So the mushroom takes some of the sugar made by the tree and in return it gives the tree vitamins and minerals and everybody lives a happy life eating a balanced diet. They did an experiment taking the fungi away from some pine saplings, and they got very sickly! Mycorrhizal mushrooms will be mostly found growing out of the ground, although they have been known to have their mycelium grow up and around a log and then grow the mushroom right out of the log, so you can be fooled.
Other mushrooms are saprophytic, meaning they eat and decay dead plant matter like tree trunks, branches, needles and leaves. So not only are mushrooms necessary for the health of trees but if it weren’t for mushrooms, fallen plant debris would not rot. Every forest would have duff so deep you wouldn’t be able to walk through it because you would sink in over your head. Some mushrooms eat the cellulose in the plants (the white squishy part) leaving the brittle brown lignin behind. These are called brown rot fungi. More difficult to do is to digest the lignin and mushrooms are some of the only organisms to evolve enzymes to be able to digest lignin (you cannot – it’s one of many reasons that wood is not considered food). These leave the white squishy cellulose behind, and are called white rot fungi. Many logs will have many different mushrooms living in them, some eating the cellulose and some eating the lignin. Sometimes you can find a piece of a rotted log that is mostly white and squishy or brown and brittle and you can see which type of fungus predominates. One study of a single log in the forest that has been going on for over 20 years has found over 200 mushrooms growing out of it so far… that’s how many different species are living there. But most astonishingly, new ones are still being discovered every year. Saprophytic mushrooms often grow right out of the piece of wood that they are eating, and can be recognized that way, but some saprophytic mushrooms just live off of the nutrients in the soil and grow up in the grass, miles away from the nearest shrub or tree. However, if there are trees nearby, there is no way to tell for sure if your mushroom sprouting out of the ground is a saprophytic or mycorrhizal mushroom.
Saprophytic mushrooms can be mass produced easily and cheaply. They grow on piles of dead things, so if get yourself a pile of dead things and sprinkle spores on it you’ll grow mushrooms. But mycorrhizal mushrooms? They need to be attached to living, sometimes old growth trees, so you can’t grow them in captivity! They have to be hunted in the wild, and that’s why they are so expensive. The health food store is not trying to rip you off because they know you love morels so much more than you love the button mushroom…. it’s because the button mushroom is saprophytic and the morel is mycorrhizal. (Well, mostly, except for the one that popped up mysteriously in your planter that one time, but that’s another story.) Every morel that you see in the store had to be found by somebody walking through the forest. And truffles grow underground, so they’re even harder to find, so the price is going to be that much higher.
The spores don’t just fall off of the mushroom, they are forcibly ejected! The mushroom wants its spores to be flung as far away as possible to spread its “seed”, so it actually launches the spores, something which will only happen when the spores are mature and ready (and their proper colour) and if the mushroom is moist enough. If your attempt to make a spore print doesn’t work it’s not that you did it wrong. It’s that the mushroom is too young (so the spores weren’t ready) or the mushroom was too old (and too dry).
Occasionally mushrooms like some Agaricus have an intermediate spore colour – the spores go from clear to pink to dark chocolate as they age. Looking at a mushroom can fool you as to the spore colour. You have to take a spore print! You might see white gills because there are no coloured spores there yet (but if you left the mushroom in the ground and watched it for a day the gills would later turn brown with spores). You might see pink gills on an Agaricus but the spores have not fully matured yet into the proper dark chocolate colour. But you can’t be fooled by a spore print. Those fake pink spores (or any young spore not fully grown and not the proper colour) will NOT fall off onto the paper! So if it is ejected onto the paper, you have a “real” spore colour. It is also most reliable to measure spores under a microscope from a spore print, not from examining a piece of tissue (especially true of Ascos) because you won’t have any small young spores confusing you and giving the wrong measurements! Squishing a piece of tissue onto a slide may actually break off spores that are not yet their full size and colour and were not yet separated from the mushroom until you came along and crushed it.
As you saw in the instructions, if two mushrooms are in the same genus it’s because they are very closely related. If they are in different genera, but they are in the same family, they are somewhat related. If they are in different classes, you know that they are only distantly related. And if they are in different phyla, you know they are just about as different as any two mushrooms can be. But, as I said, there are only 6 levels of fungi in this classification system. For instance, Hygrocybe and Hygrophorus and Chrysomphalina are all genera in the Hygrophoraceae family. Hygrocybe and Hygrophorus are more closely related to each other (I think), but there’s no way for you to know that. You would need to add a new level, a sub-family (or a super-genus, not to be confused with Wile E. Coyote Super-Genius) to the tree to show that relationship. People have created many different sub-levels to show more detail, but it’s never going to be perfect until you have an infinite number of levels, which just isn’t practical. So this system, like any man-made system, is just a flawed attempt to show at least some of the relationships between mushrooms. You need a picture of a full phylogenetic tree to show the exact relationships between all the mushrooms, but we won’t get into that here.
Another interesting philosophical argument is: What is a species? OK, so you go through the keys and you find the mushroom you have, and you are able to put a name on it. Is that the mushroom species that you have? Maybe not. The field of mycology started out in Europe, where people went around and named all of the mushrooms they found. Then later, in North America, people started going around looking at all the mushrooms here, first of all along the east coast. They noticed that some mushrooms looked the same as the ones in Europe and some were different, so they used the European names for the ones that looked the same over here and made up new names for the new ones. Then they started looking on the west coast and they noticed that some were the same as in Europe, some were the same as on the east coast and some were new. Except that is not necessarily true. The Amanita muscaria out west sure looks like the same one found in Europe for thousands of years, but a closer analysis shows that it just might be genetically different enough to perhaps be a different species. Some mycologists want to call it “Amanita amerimuscaria”. Once a mushroom migrates over here and gets isolated from its original population it will start to evolve and drift from the original European mushroom. Eventually there is enough difference that it becomes a new species. And there may be a lot of changes, but to the human eye it still looks the same, so sometimes you can’t actually tell which species you have without doing DNA analysis. But that’s not the final word either. No two mushrooms are alike, and no two people are alike. How far apart do two mushrooms have to be before they are considered a different species? Believe it or not, there is no generally accepted answer to that question. Some people think that if two organisms can mate with each other and produce viable offspring (that themselves are capable of reproducing) then they are the same species, and if they can’t, then they are not. That is called a “biological species”. However, sometimes it is not possible to test this. Some people say “if the DNA is more than 1% different, let’s call it a different species” but that is arbitrary. Some people say “if we find a whole bunch of mushrooms very much like each other and a second bunch of mushrooms all like each other, but we can’t find any mushrooms that are in between, let’s call them two different species.” But then what happens when you later find a mushroom that’s right in between? You have to change your mind and say “I guess they weren’t separate species after all”. This has happened.
So the current state of affairs is that the DNA work is starting to be done to see if the thousands of mushrooms named in Europe are really the same thing over here. Somebody is going to make a judgment call (there are no right or wrong answers) and if the mushroom is “different enough” they will give the North American mushroom a different name and say that it is a different mushroom, even though to humans, they look the same. For instance, Helvella lacunosa, the fluted elfin saddle just got a new name here in the PNW – Helvella vespertina. It looks the same in Europe as it does here (although some people argue there are tiny differences) but if you find it in Europe you are supposed to call it “Helvella lacunosa” and if you find it in Seattle you are supposed to call it “Helvella vespertina”. Its DNA is different over here. Different enough that somebody thought it deserved a different name. Perhaps the only objective way to answer these questions is to choose the definition of “biological species”, but it takes a long time to do those studies, much longer than it takes to sequence some DNA, so that work is not going to be done anytime soon. Nor can everybody agree that that is how it should be done.
To make things official, every mushroom is supposed to have a “type”, the first mushroom found like it, which you are supposed to keep in a museum. You can say with absolute certainty that the first Helvella lacunosa found in 1783 in Europe is properly called Helvella lacunosa. But what about the millions of other mushrooms just like it that have been found since? Are they also Helvella lacunosa? Now you understand that some people would say yes and some people would say no. The problem is, we didn’t save the original specimens until recently, so there is no original “type” for Helvella lacunosa and many other common mushrooms first named long ago (we didn’t start saving them until more recently, and even if we did save them they’re so old now that you can’t always extract DNA). So you will never be able to prove it one way or another. For all such mushrooms somebody has to go back to the general area where the original one was found, find something as close to it as they possibly can, and declare that one the new “type” (and then remember to save it this time.)
Over the years, as we try and figure out which mushrooms are related to each other, we’ve gotten better and better at figuring that out and we have changed the names of the mushrooms many times to try and express that. Most mushrooms have many synonyms, alternative Latin names you could use for the mushroom, and not everybody agrees on which name is the right one. Some have dozens of possible names! So you will see the same mushroom on these pages called something different by a different book or a different person. The right name will continue to be argued over until we can say with certainty which other mushrooms it is related to and also say with certainty who named it first and therefore has priority.
Many of the guidebooks you will find, such as Mushrooms Demystified, were written a long time ago and DNA studies have shown that mushrooms are not related to each other in the ways we used to think. If David were to rewrite that book now, the chapters would be organized differently (and he just might.) The reason a couple of the chapters are so big is that only the most distinctive mushrooms got their own family (or chapter) back in the day, and everything left over was placed in a miscellaneous family (sometimes called the garbage family). It took years of microscopic and molecular study to figure out the differences between these leftover mushrooms and to create families where each mushroom can rightfully belong. And this work is still ongoing! It will be years before we have the answers. Mushrooms are being renamed and moved around the tree of life every month! We live in interesting times, for it may be true that sooner than we think these questions will be answered once and for all and so we can dream that one day our children will not have to live in a world where mushroom names are being changed all the time.
Now I bet there is one question going through many people’s minds right now… “Who cares?!?”. As humans we love to categorize things, but the level of detail we choose to categorize things to should depend on whether or not declaring two mushrooms the same or different is actually useful somehow. Perhaps it is your job to trace how mushrooms have evolved and study how long it takes an organism to develop significant differences after being introduced to an isolated island. Then you absolutely want to try and figure all of this out. Is one species going extinct but a closely related species thriving and you are trying to figure out what changes are happening in the environment and how it might affect us? Then you also care. But if you just want to learn to recognize and enjoy the beautiful mushrooms around you, maybe you are completely content knowing that you have narrowed the identity of one down to a closely related group of species. What if you just want to eat it? You usually won’t care, but it turns out that the popular edible mushroom Macrolepiota rachodes turned out to be three different species, and the popular honey mushroom Armillaria mellea turned out to be nine different species all hiding in a species complex that looked almost identical. They differ by very little, except that some people are allergic to some of them (and get sick eating them) but not others. Now that somebody did the work of sorting out the minute differences that make up the different species (work which to some I’m sure seemed pointless), these people can figure out reliably which ones they can eat!
One very interesting thing we learned as we started to delve into the true relationships of the mushrooms is that there were some big surprises of mushrooms that looked alike but turned out to be completely unrelated as well as mushrooms that couldn’t look more different that turned out to be closely related. For instance, many puffballs and the little bird’s nest fungi turned out to be related to the store bought Agaricus mushroom. Yet Russula and Lactarius, although looking for all the world like every other gilled mushroom, are not closely related to any other gilled mushroom at all. It turns out that there are only so many ways you can look to be successful in life (if you’re a mushroom). You need to maximize the surface area to volume ratio of your spore-bearing hymenium, which simply means that you need to make as many spores as possible if you hope to reproduce. While more “primitive” mushrooms only make spores on the surface of a piece of wood, eventually more “clever” mushrooms evolved to produce a wrinkled surface instead of a flat surface, in order to have more room to make more spores in and around each of the folds. Eventually, gills evolved, where the face of each gill is coated in spores, much like the pages of a book, producing thousands of times more spores than simply coating a flat surface would. Another strategy is to grow pores, like the boletes and polypores do. They evolved tubes (like a bundle of straws you hold in your hand) where the inside of each tube is coated with millions of spores. A third strategy is to develop spines (also called teeth), kind of like inside-out pores where the surface of each spine can be coated in many, many spores. These three shapes are the most successful for reproducing and they have evolved independently over and over again, seemingly by coincidence, so that we now have mushrooms that look identical but are millions of years apart in evolution. Except now we understand that it isn’t really coincidence – when you get into a harsh environment and are pressured to evolve more spores or go extinct, you find a way to evolve into one of those three shapes. This is called convergent evolution.
The shapes of Gastroid and Truffle-like fungi especially have evolved independently many, many times, as explained on the truffle page.
So that begs the question, what is a gilled mushroom? The answer used to be easy – anything with gills. But as we started to make up the mushroom family tree to accurately represent their relationships to each other we now have mushrooms with gills all over the tree and there is no longer just one branch of the tree with gills. You might choose to define a gilled mushroom as everything related to the store button mushroom Agaricus (technically the Order Agaricales) which was the first mushroom ever named and therefore gets to be the official gilled mushroom. But this means that some mushrooms with gills (like some polypores and Russulas) are not gilled mushrooms, but puffballs and bird’s nests are gilled mushrooms. You can see why this might be controversial.
Edibility is another controversial subject. Mushrooms are very hard to identify and at first, you are not going to get the identity of most mushrooms correct when you use these pages (or any other book or key). And if you eat the mushroom, you might kill yourself. Identifying correctly is very hard to do and can only be done after getting a lot of hands-on experience with a trained teacher. There are many things that can go wrong
- There are over 5,000 mushrooms in the PNW and most books only list a few hundred, so your mushroom is probably not even in your book.
- Different mushrooms can look almost identical and it takes a trained eye to spot the differences.
- Identifying is hard enough in person – via a photograph it’s even harder still.
- Every key and book I know has mistakes in it, including this one, sometimes giving you the wrong information and sometimes having the wrong photograph for a certain species.
- I am always finding mushrooms that grew under odd circumstances and look nothing like they normally do, often looking much like a different mushroom.
- Mushrooms change a lot as they age, and a photograph can only show one at one point in its life cycle.
- Where you live, there may be deadly poisonous mushrooms that don’t grow elsewhere and look like edible mushrooms where your guidebook was written, so the guidebook might not warn you about them.
- Many people are allergic to mushrooms and get sick eating things that others can eat perfectly well. So always try only a small piece of a new confirmed edible species and only eat more of it if you don’t feel sick after an hour or so.
I’m not trying to discourage you from learning. With enough practice you can learn to identify hundreds of different mushrooms from blurry photographs, but it takes years. Go ahead and try and identify all the mushrooms you find, and use the result as the starting point for learning more about that species and how to identify it, but just in case you’re wrong, don’t eat it.
There are a lot of common “rules” floating about that say things like “Do not eat a bolete that turns blue wherever it is touched”, or “poisonous mushrooms are white or have red pores” but none of these rules are true. A rule like this comes about because somebody discovers a poisonous mushroom that turns blue, or is white, or has red pores, so they make a rule saying not to eat mushrooms that have that property. But the truth is, there are probably 99 edible mushrooms that are white or turn blue for every one that is poisonous, so it’s not a very good rule. You might think that at least it’s a good “better safe than sorry” rule, except that there are deadly poisonous mushrooms that don’t look like any of the mushrooms in any of the rules, so you can needlessly avoid hundreds of good edible species and only eat a mushroom that doesn’t follow any of the “bad” rules, and you might still die. So much for rules.
OK, this is the moment you’ve all been waiting for. I am about to tell you the real way to tell if a mushroom is edible or not… are you ready? Here goes… you eat it and then see what happens to you.
Yes, here we are well into the 21st century, and there is still no better way. There may be hundreds of different toxins in different mushrooms, and we don’t even know what they are, so we can’t make a test for them. Sure, whenever a mushroom kills somebody it gets funding, and we learn why. So there is a test for amatoxin, the deadly poison in Amanita phalloides and other mushrooms, but for the vast majority of poisons out there there is no test. So every time you read in a guidebook “edible, edible, poisonous, edible” it’s because some brave person long ago ate them and passed them around to a few of their equally brave (or naively trusting) friends, and wrote down which ones made them sick, and how sick they got. Then, as mushrooms started killing people, we found fewer brave volunteers to try all the unknown and newly discovered mushrooms, which is why in more recent guidebooks describing more recently discovered mushrooms, you’ll see a whole lot of “unknown, don’t know, unknown, no idea”.
I think it is true to say that there are far fewer poisonous mushrooms than we think. If anybody got scared or felt weird when eating a mushroom, nobody else would be brave enough to try it, so a lot of the mushrooms received bad reputations for no good reason. Many “poisonings” were allergic reactions that you or I might not have if we ate it. And if the first person ate it and was fine but the second person ate it and got sick, we wrote “edible, but use caution as some people can’t tolerate it”. But if the first person got sick and the second person was fine we wrote “poisonous but not to everybody”. Same edibility. But it is still true that some mushrooms can kill you, so no matter how few of them are actually poisonous, don’t eat something you can’t ID with 100% accuracy, because you might get unlucky.
Don’t trust what somebody else says unless you personally know their credentials. All the time I see people on the internet post pictures and ask what a mushroom is, and I see other people respond naming it as a species that is edible, and they are wrong. Sometimes they even admit they’re only guessing because they’re only trying to learn themselves and hoping that somebody will tell them if they are right or wrong. They don’t expect the other person to eat it based on their guess. But some people seem to trust a stranger on the internet whom they have never met with their life, and they will eat it. Remember, that is what you are doing, trusting that person whom you’ve never met with your life.
If you hang around with an identifier long enough you’ll notice that they are always finding strange mushrooms in the woods and saying “Hmm, I wonder what that is?” and popping them in their mouth. What is going on? Are they trying to kill themselves? Well, some mushrooms that look alike only differ by taste, so you have to taste them to find out what mushroom you have. As long as you spit them out, barring bacteria and environmental toxins, you’re probably OK. The poisons are long chain proteins usually too large to be absorbed through the mucous membrane of the roof of your mouth – you would have to actually swallow a piece to get poisoned. You might not want to go around chewing the deadly Amanita just on principle. Mycologists have a pretty good idea that their mushroom is not deadly poisonous before they risk a taste, but my point is that you should not be afraid to touch a mushroom. And there is certainly no reason to avert your children’s eyes away from the forest for fear that they see a poison mushroom and it hurt them.
So in summary, if you would like to start eating wild mushrooms, my advice to you is to find an expert who can teach you, in person, just a few of your favourite edibles and their poisonous lookalikes, and then practice with that person until they are confident that you can do it on your own. Since there are no general rules to identify edible mushrooms, you will have to start with a small number of species, non-gilled if you want to be safest (since they are easier to identify and fewer of them are poisonous), and learn all the lookalikes from a real person. Don’t try to do it from any book or publication.
Microscopy
If you are just starting out, you are probably not yet thinking of getting a microscope to look at mushrooms for identification, but eventually you will need to. A macroscopic key can only get you so far. You might notice a number of species are described very similarly on these pages, and are wondering how you can learn to tell them apart. The answer is that you probably can’t without a microscope and more information on what to look for. Subtle differences are not usually reliable, so trying to learn to identify lookalike species by studying their tiny differences is misguided – it really might be either one.
Here is some advice on how to get started with a microscope, when you’re ready. Books will tell you many, many things you can look for under the scope to identify your mushroom, but they won’t tell you how difficult they are to find. You can see most everything you need to see with a good 400 power system. Don’t feel you need to spend the extra money right away for a 1000 power oil lens. You can see the shape and size of spores and measure them to within a micron or two. Don’t expect at first to be able to tell the difference between species whose spore sizes differ by less than that. Most of the time, you will see if the spores are smooth or have some kind of warts, but not always. Telling Basidiomycota from Ascomycota is very easy to do. So is finding odd shaped cystidia.
However, if you are told to look for clamp connections or tell the difference between monomitic and dimitic or trimitic hyphae, do not expect to be able to do that without a very good quality 1000 power lens and a whole lot of practice and patience. The same goes for trying to find the structure of the cap and gills. If you know what you can realistically find as a beginner, you will save yourself from getting frustrated and impatient.
I’d love to be able to answer all of your questions, but the unfortunate fact is that there is so much about mushrooms that we just don’t know. Mycology does not get a lot of funding, and there are not a lot of people working in the field compared to botany, mammalogy and, well, just about every other part of biology except for slime molds. (Even mycologists feel sorry for slime molds in the Kingdom Protista and sometimes study them out of pity). There is just so much we don’t know and are not likely to learn anytime soon, which is unfortunate considering that everything we do know is telling us how crucial fungi are to every part of the life cycle on this planet. The mystery is one reason we find them so fascinating and amateur enthusiasts from mushroom clubs are often able to help professional mycologists in many ways. Not only are they fun to study, but it’s great to know that what you do can make a difference! The field needs all of the assistance it can get.
Don’t feel bad if you have trouble matching a mushroom you find to the pictures on these pages. Individual mushrooms of a species can vary tremendously. Imagine you are an alien that has come to Earth and you say “take me to your leader” so they bring you to President Obama. Now you’re wondering which species he is, so you consult your guidebook to creatures of the Milky Way galaxy, and the picture they have under “human” is a photo of Prince William’s new baby. You would go back to your home planet and swear up and down that you couldn’t possibly have spoken to a human. Read and become familiar with as many references as you can, and make sure to note the variety each individual can demonstrate every time you find a mushroom, and don’t give up.