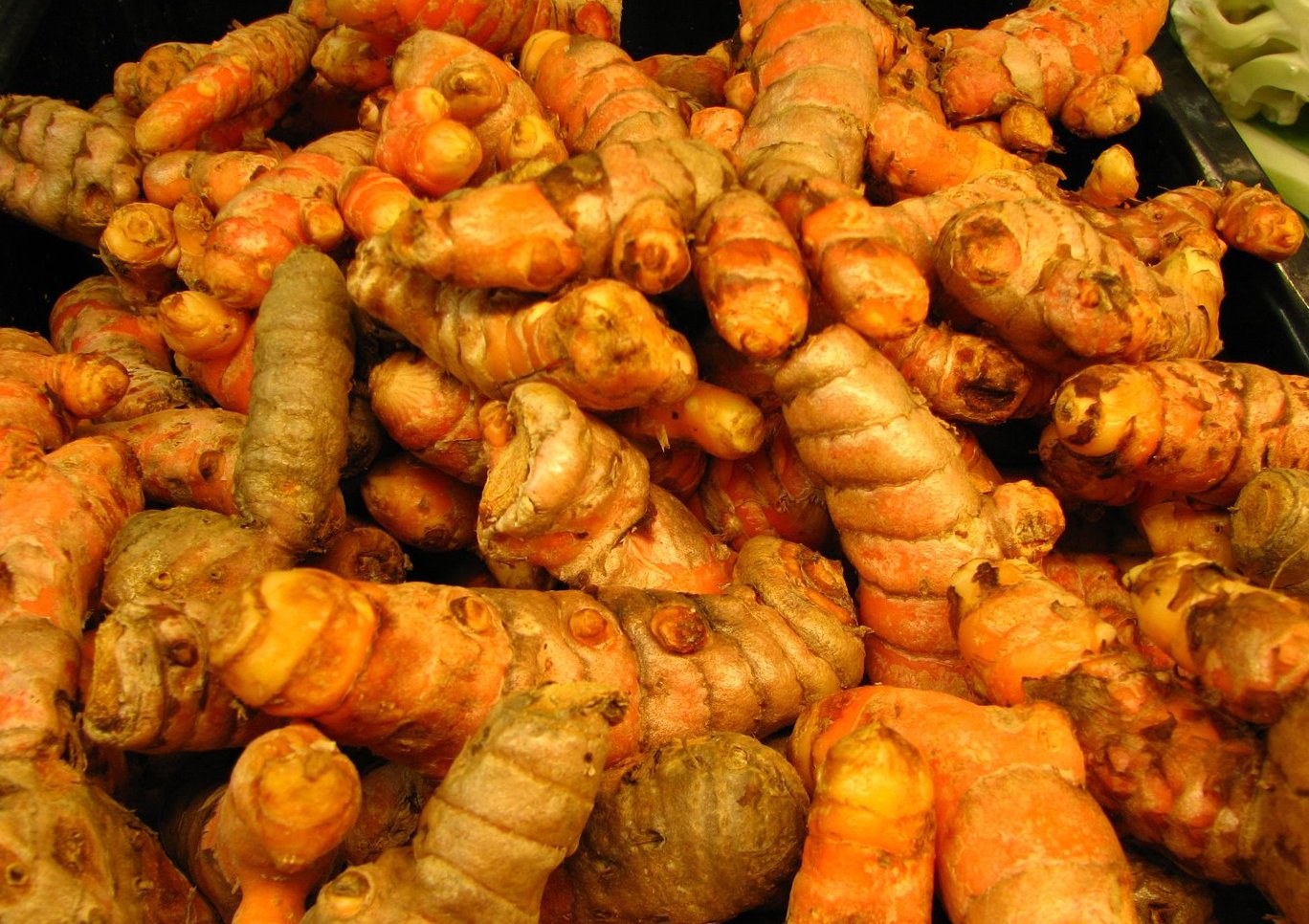
We have all heard of taking turmeric (curcumin) with black pepper (piperine) to enhance the bioavailability and absorption of curcumin. Piperine, the bioactive compound found in black pepper, has been shown to significantly increase the absorption of curcumin by inhibiting metabolic enzymes that break it down and increasing intestinal permeability.
Curcumin is a highly effective anti-inflammatory agent valuable in managing inflammatory conditions like arthritis, inflammatory bowel diseases, and certain cancers by blocking inflammatory molecules like cytokines and enzymes like cyclooxygenase-2 (COX-2), a potent antioxidant capable of neutralizing free radicals and reducing oxidative stress, a major contributor to aging and chronic diseases like Alzheimer’s and Parkinson’s, where it may help protect against age-related conditions by scavenging free radicals and boosting the body’s antioxidant defenses, and exhibits anti-cancer properties by inhibiting tumor growth, metastasis, angiogenesis, and inducing apoptosis in cancer cells while leaving healthy cells unharmed, with numerous studies exploring its potential in preventing and treating various cancers, including breast, prostate, colon, and lung cancer.
Piperine is not an emulsifier. Piperine and emulsifiers are different types of compounds that enhance bioavailability through different mechanisms. Emulsifiers are substances that help stabilize mixtures of two immiscible liquids, such as oil and water, by preventing them from separating into distinct layers. They work by surrounding oil droplets with a protective layer, increasing their surface area, and making it easier for digestive enzymes and bile salts to break them down, facilitating the absorption of fat-soluble nutrients or compounds.
Black pepper that enhances bioavailability primarily through two mechanisms:
- Inhibiting metabolic enzymes: Piperine inhibits certain enzymes like glucuronidation enzymes that are responsible for breaking down and eliminating compounds like curcumin from the body. By inhibiting these enzymes, piperine allows more of the compound to remain intact and available for absorption.
- Increasing intestinal permeability: Piperine has been shown to increase the permeability of the intestinal lining, allowing for better absorption of compounds like curcumin into the bloodstream.
However, if someone has a leaky gut or increased intestinal permeability, there are some important considerations regarding the use of piperine. While piperine can enhance nutrient absorption by increasing intestinal permeability, this mechanism may exacerbate an already compromised gut barrier in cases of leaky gut. With increased intestinal permeability, there is a higher risk of undigested food particles, toxins, and other antigenic substances entering the bloodstream, potentially triggering an immune response and inflammation. Furthermore, piperine may also facilitate the absorption of harmful substances, such as bacterial endotoxins or environmental toxins, which could further contribute to inflammation and gut dysbiosis.
IIn such cases, it may be advisable to avoid or limit the use of piperine until the gut barrier is repaired and intestinal permeability is improved. Instead, the focus should be on natural gut-healing strategies, such as eliminating inflammatory foods, consuming gut-supportive nutrients from sources like bone broth (which provides collagen peptides and glutamine), pumpkin seeds (zinc), sweet potatoes (vitamin A), and mushrooms (vitamin D), and incorporating probiotic-rich foods like yogurt, kefir, and fermented vegetables.
Also, one can consider using alternative bioavailability enhancers that do not increase intestinal permeability, such as emulsifiers (lecithin from soy or sunflower), lipid carriers, or absorption-enhancing enzymes like bromelain from pineapples (core) and papain from papaya. Consider making a powerful juice!
The goal should be to first restore a healthy gut barrier and reduce intestinal permeability through natural means, after which piperine may be reintroduced cautiously to improve nutrient absorption as part of a comprehensive terrain therapy approach.
Emulsifiers possess a unique molecular structure with both hydrophilic (water-loving) and lipophilic (fat-loving) regions, allowing them to interact with both water and oil molecules simultaneously. When introduced into a mixture of oil and water, emulsifiers form stable emulsions by surrounding the oil droplets with a protective layer, preventing them from coalescing and separating from the water phase. This emulsification process significantly increases the surface area of the oil droplets, making it easier for digestive enzymes, such as lipases and bile salts, to break them down and facilitate the absorption of the fat-soluble nutrients or compounds they contain.
Emulsifiers serve as effective “medicinal transfer agents,” facilitating the absorption and delivery of beneficial compounds like curcumin into the body. Just as piperine enhances curcumin’s bioavailability, emulsifiers play a crucial role in improving the solubility and dispersibility of fat-soluble nutrients and lipophilic compounds in the digestive tract. By forming stable emulsions and increasing the surface area of oil droplets, emulsifiers promote better digestion and absorption of these beneficial substances. Lecithin, phospholipids, and liposomes are common emulsifiers used in supplements and functional foods for this purpose.
IInterestingly, cacao (cocoa) butter, the natural fat found in pure chocolate, possesses emulsifying properties due to its unique composition of fatty acids and phospholipids. This characteristic makes cacao a potential natural emulsifier that may enhance the absorption of fat-soluble nutrients and medicinal compounds when consumed alongside them. This is one reason why I like to incorporate medicinal herbs into pure cacao preparations, as the cacao butter can act as a natural emulsifier, potentially improving the bioavailability and delivery of the beneficial compounds found in those herbs.
Emulsifiers help our bodies absorb and use fat-soluble nutrients and compounds better. They do this in two main ways. First, emulsifiers can mix with bile salts, which are substances released by the liver into the small intestine to help digest and absorb fats and fat-soluble things. When emulsifiers and bile salts combine, they form tiny structures called mixed micelles. These mixed micelles can wrap around and carry fat-soluble nutrients and compounds, helping them move across the intestinal wall and enter the bloodstream more easily.
Secondly, some emulsifiers can interact with special transporters found on the cells lining the intestine. These transporters are like little doorways that allow nutrients and compounds to pass through the intestinal wall and into the bloodstream. By interacting with these transporters, emulsifiers can help fat-soluble substances that they have dissolved or encapsulated to be taken up and absorbed more efficiently. For example, emulsifiers like lecithin and phospholipids may interact with transporters that are responsible for absorbing fats, vitamins, and other fat-soluble things, potentially increasing their absorption.
In simple terms, emulsifiers not only help dissolve and disperse fat-soluble nutrients and compounds in the digestive system but also actively assist in their journey across the intestinal barrier. This happens through the formation of mixed micelles that act as carriers and by interacting with special transporters on intestinal cells, ultimately leading to better absorption and use of these beneficial fat-soluble substances in the body.
Common emulsifiers used for enhancing bioavailability include lecithin (derived from soy or sunflower), phospholipids (such as phosphatidylcholine and phosphatidylserine), and liposomes (spherical vesicles made of phospholipids). These emulsifiers can be found in supplements, functional foods, and pharmaceutical formulations designed to improve the solubility, dispersibility, and ultimately, the absorption and bioavailability of fat-soluble nutrients and lipophilic compounds like curcumin.
While focusing on gut-healing strategies like eliminating inflammatory foods, consuming gut-supportive nutrients from natural sources like bone broth (glutamine), pumpkin seeds (zinc), sweet potatoes (vitamin A), and mushrooms (vitamin D), and incorporating natural supplements like l-glutamine, collagen peptides, and probiotic-rich foods like yogurt, kefir, and fermented vegetables, you can incorporate emulsifiers to enhance curcumin’s bioavailability. The goal should be to first restore a healthy gut barrier and reduce intestinal permeability through natural means, after which piperine may be reintroduced cautiously to improve nutrient absorption as part of a comprehensive terrain therapy approach.
Turmeric-Cacao Anti-Inflammatory (emulsifier) Chocolate
Ingredients
– 1/2 cup cacao butter or cocoa butter
– 1/4 cup cacao paste (or 100% unsweetened chocolate)
– 2 tablespoons ground turmeric
– 2 tablespoons MCT coconut oil (acts as an emulsifier)
– 2 tablespoons raw honey
– 1 teaspoon ground ginger
– 1/2 teaspoon ground cinnamon
– 1/4 teaspoon cayenne pepper (or more to taste)
– 1/4 teaspoon sea salt
– 1 tablespoon hemp seeds (optional)
Instructions
- In a double boiler or heat-safe bowl set over a pot of simmering water, gently melt the cacao/cocoa butter and MCT coconut oil, stirring occasionally until completely liquid.
- Remove from heat and whisk in the cacao paste, turmeric, honey, ginger, cinnamon, cayenne pepper, and sea salt until well combined and smooth.
- If using, stir in the hemp seeds.
- Pour the mixture into silicone molds or onto a parchment-lined baking sheet and spread it out evenly.
- Refrigerate for at least 2 hours or until completely set.
- Once set, pop the chocolate out of the molds or break it into pieces if poured onto a sheet.
- Store in an airtight container in the refrigerator for up to 2 weeks.
This anti-inflammatory, gut-healing chocolate features several powerful ingredients. The cacao butter and MCT coconut oil act as natural emulsifiers, potentially enhancing the absorption of the anti-inflammatory turmeric. Ginger and cinnamon provide additional anti-inflammatory benefits, while cayenne pepper offers a subtle kick of heat and may also have anti-inflammatory properties. Hemp seeds (optional) add a nutty flavor and a boost of plant-based protein and healthy fats.